Battery technology is crucial in various applications, particularly in consumer electronics, electric vehicles, and grid-scale storage. For large-scale applications, it’s feasible to enhance battery lifespan by modifying chemistry and optimizing charging cycles.
However, consumer electronics often rely on smaller, lightweight batteries, which face quicker degradation due to rapid charging demands. As a result, these batteries can show significant performance drops after just a few years of use, primarily due to internal fragmentation within the electrode materials, which leads to a loss of usable lithium.
Recent research has indicated a potential solution to this problem, particularly for lithium-silicon batteries. These batteries utilize silicon anodes, allowing for a higher density of lithium storage compared to traditional materials. However, the drawback is that the silicon particles expand significantly when lithium is stored, leading to fragmentation and a subsequent decline in capacity.
Some companies are already addressing this issue by limiting the amount of silicon or designing around its expansion, but pure lithium-silicon batteries are less common and often face challenges due to this fragmentation.
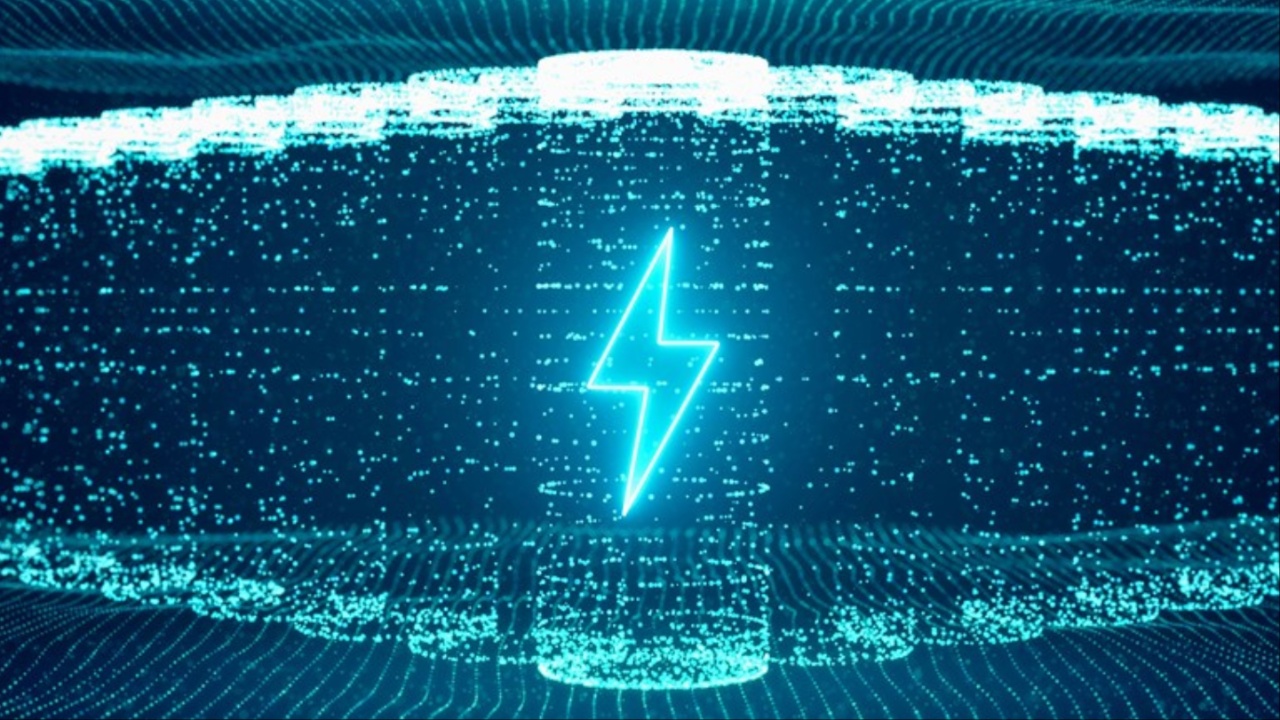
Stanford Researchers Discover Method to Restore Capacity in Fragmented Lithium-Silicon Batteries, Extending Lifespan
Researchers at Stanford University have proposed a novel approach to address the fragmentation issue. They hypothesized that it might be possible to re-establish connections between fragmented silicon particles and the battery’s electrical system, effectively restoring lost capacity.
This was based on the understanding that fragmented silicon, despite being disconnected, could still be influenced by electric fields due to its uneven charge distribution. Their experiments demonstrated that by applying a voltage to a solution containing these fragments, they could attract the particles back to the electrode, thus re-establishing their connection to the battery’s charge management system.
The findings from the study were promising, showing that the application of four volts for five minutes could lead to significant capacity restoration. After performing around 20 charge/discharge cycles, researchers noted a capacity increase of over 30%.
When the fragmented electrodes underwent 200 cycles before treatment, the capacity more than doubled, achieving a 140% recovery. These results suggest that the reconnected fragments behave similarly to the new material, providing an effective strategy to extend battery life.
While the initial results are encouraging, they raise questions about the broader applicability of this method. The current focus on silicon anodes leaves open the potential for similar techniques to be adapted for other materials that exhibit fragmentation.
However, it is less likely to be effective with traditional graphite electrodes. The research points to an intriguing direction for future battery technology, especially considering that Yi Cui, one of the authors, has experience in developing lithium-silicon batteries, hinting at the possibility of applying this restoration technique to other battery types as well.
